ICU Management & Practice, Volume 18 - Issue 3, 2018
Mechanisms of endotoxin-induced multi-organ damage.
Endotoxins as PAMPs
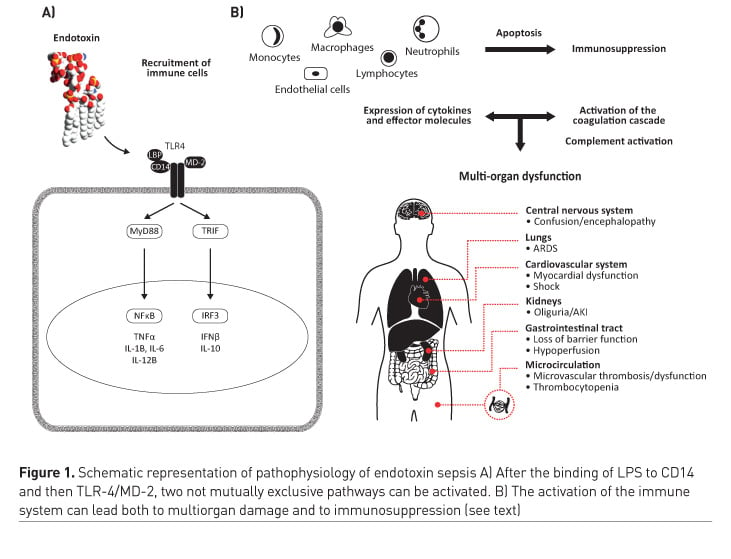
Clinical relevance of LPS
Pathophysiology of organ damage in Gram-negative sepsis
Endotoxin and the immune system
Therapeutical approach
Conclusion
Conflict of interest
Abbreviations
ADP action potential duration
AKI acute kidney injury
ALI acute lung injury
DAMP damage-associated
molecular pattern
EAA endotoxin activity assay
GFR glomerular filtration rate
ICU intensive care unit
LPS lipopolysaccharide
PAMP pathogen-associated molecular pattern
PRR pattern recognition receptor
RCT randomised controlled trial
REA reactive oxygen species
TLR toll-like receptor
TRIF toll-like receptor domain adaptor inducing interferon-β
References:
Cantaluppi V, Assenzio B, Pasero D et al. (2008) Polymyxin-B hemoperfusion inactivates circulating proapoptotic factors. Intensive Care Med, 34(9): 1638-45.
Chagnon F, Bentourkia M, Lecomte R et al. (2006) Endotoxin-induced heart dysfunction in rats: assessment of myocardial perfusion and permeability and the role of fluid resuscitation. Crit Care Med, 34(1): 127-33.
Chagnon F, Metz CN, Bucala R et al. (2005) Endotoxin-induced myocardial dysfunction: effects of macrophage migration inhibitory factor neutralization. Circ Res, 96(10): 1095-102.
Cohen J, Cristofaro P, Carlet J et al. (2004) New method of classifying infections in critically ill patients. Crit Care Med, 32(7): 1510-26.
Cohen J (2000) The detection and interpretation of endotoxaemia. Intensive Care Med, 26 Suppl 1: S51-6.
Cruz DN, Antonelli M, Fumagalli R et al. (2009) Early use of polymyxin B hemoperfusion in abdominal septic shock: the EUPHAS randomized controlled trial. JAMA, 301(23): 2445-52.
Cunningham PN, Dyanov HM, Park P et al. (2002) Acute renal failure in endotoxemia is caused by TNF acting directly on TNF receptor-1 in kidney. J Immunol, 168(11): 5817-23.
Cutuli SL, Artigas A, Fumagalli R et al. (2016) Polymyxin-B hemoperfusion in septic patients: analysis of a multicenter registry. Ann Intensive Care, 6(1): 77.
Danner RL, Elin RJ, Hosseini JM et al. (1991) Endotoxemia in human septic shock. Chest, 99(1): 169-75.
de Grooth HJ, Postema J, Loer SA et al. (2018) Unexplained mortality differences between septic shock trials: a systematic analysis of population characteristics and control-group mortality rates. Intensive Care Med, 44(3): 311-22.
Delano MJ, Ward PA (2016) Sepsis-induced immune dysfunction: can immune therapies reduce mortality? J Clin Invest, 126(1): 23-31.
Marshfield E (2011) Endotoxin tolerance and the immune system. Plymouth Student Scientist, , 4, (2), 242-51.
Esteban E, Ferrer R, Alsina L et al. (2013) Immunomodulation in sepsis: the role of endotoxin removal by polymyxin B-immobilized cartridge. Mediators Inflamm, 2013: 50739.
Flesch M, Kilter H, Cremers B et al. (1999) Effects of endotoxin on human myocardial contractility involvement of nitric oxide and peroxynitrite. J Am Coll Cardiol, 33(4): 1062-70.
Ilia S, Briassoulis P, Briassoulis G (2017) Polymyxin B hemoperfusion in septic shock: nothing overmuch (Meden Agan)! J Thorac Dis, 9(9): 2716-9.
Klein DJ, Derzko A, Foster D et al. (2007) Daily variation in endotoxin levels is associated with increased organ failure in critically ill patients. Shock (Augusta, GA), 28(5): 524-9.
Klein DJ, Foster D, Schorr CA et al. (2014) The EUPHRATES trial (Evaluating the Use of Polymyxin B Hemoperfusion in a Randomized controlled trial of Adults Treated for Endotoxemia and Septic shock): study protocol for a randomized controlled trial. Trials, 15: 218.
Lancel S, Joulin O, Favory R et al. (2005) Ventricular myocyte caspases are directly responsible for endotoxin-induced cardiac dysfunction. Circulation, 111(20): 2596-604.
Levi M, Sivapalaratnam S (2018) Disseminated intravascular coagulation: an update on pathogenesis and diagnosis. Expert Rev Hematol, 11(8): 663-72.
Marshfield E (2011) Endotoxin intolerance and the immune system. Plymouth Student Scientist, 4(2): 242-51.
Marshall JC, Foster D, Vincent JL et al. (2004) Diagnostic and prognostic implications of endotoxemia in critical illness: Results of the MEDIC study. J Infect Dis, 190(3): 527-34.
Matute-Bello G, Downey G, Moore BB et al. (2011) An official American Thoracic Society workshop report: features and measurements of experimental acute lung injury in animals. Am J Respir Cell Mol Biol, 44(5): 725-38.
McIntyre CW, Harrison LE, Eldehni MT et al. (2011) Circulating endotoxemia: a novel factor in systemic inflammation and cardiovascular disease in chronic kidney disease. Clin J Am Soc Nephrol, 6(1): 133-41.
Mogensen TH (2009) Pathogen recognition and inflammatory signaling in innate immune defenses. Clin Microbiol Rev; 22(2): 240-73.
Molteni M, Gemma S, Rossetti C (2016) The Role of Toll-Like Receptor 4 in Infectious and Noninfectious Inflammation. Mediators Inflamm, 6978936.
Monti G, Bottiroli M, Pizzilli G et al. (2010) Endotoxin activity level and septic shock: a possible role for specific anti-endotoxin therapy? Contrib Nephrol, 167: 102-10.
Morrell ED, Kellum JA, Pastor-Soler NM et al. (2014a) Septic acute kidney injury: molecular mechanisms and the importance of stratification and targeting therapy. Crit Care, 18(5): 501.
Morrell ED, Kellum JA, Hallows KR et al. (2014b) Epithelial transport during septic acute kidney injury. Nephrol Dial Transplant, 29(7): 1312-9.
Nakano D, Doi K, Kitamura H et al. (2015) Reduction of tubular flow rate as a mechanism of oliguria in the early phase of endotoxemia revealed by intravital imaging. J Am Soc Nephrol, 26(12): 3035-44.
Opal SM, Gluck T (2003) Endotoxin as a drug target. Crit Care Med, 31(1 Suppl): S57-64.
Opal SM, Scannon PJ, Vincent JL et al. (1999) Relationship between plasma levels of lipopolysaccharide (LPS) and LPS-binding protein in patients with severe sepsis and septic shock. J Infect Dis, 180(5): 1584-9.
Opal SM (2010) Endotoxins and other sepsis triggers. Contrib Nephrol, 167: 14-24.
Opal SM (2007) The host response to endotoxin, antilipopolysaccharide strategies, and the management of severe sepsis. Int J Med Microbiol, 297(5): 365-77.
Payen DM, Guilhot J, Launey Y et al. (2015) Early use of polymyxin B hemoperfusion in patients with septic shock due to peritonitis: a multicenter randomized control trial. Intensive Care Med, 41(6): 975-84.
Peng X, Hassoun PM, Sammani S et al. (2004) Protective effects of sphingosine 1-phosphate in murine endotoxin-induced inflammatory lung injury. Am J Respir Crit Care Med, 169(11): 1245-51.
Proudfoot AG, McAuley DF, Griffiths MJ et al. (2011) Human models of acute lung injury. Dis Model Mech, 4(2): 145-53.
Reinhart K, Daniels R, Kissoon N et al. (2017) Recognizing sepsis as a global health priority - a WHO resolution. N Engl J Med, 377(5): 414-7.
Rhodes A, Evans LE2, Alhazzani W et al. (2017) Surviving Sepsis Campaign: International Guidelines for Management of Sepsis and Septic Shock: 2016. Intensive Care Med, 43(3): 304-77.
Rodriguez-Gonzalez R, Ramos-Nuez A, Martin-Barrasa JL et al. (2015) Endotoxin-induced lung alveolar cell injury causes brain cell damage. Exp Biol Med (Maywood), 240(1): 135-42.
Romaschin AD, Klein DJ, Marshall JC (2012) Bench-to-bedside review: clinical experience with the endotoxin activity assay. Crit Care, 16(6): 248.
Saha R, Das S, Chatterjee R et al. (2010) The pathophysiology of septic shock. Int J Pharma Bio Sciences, 1(12): 1-10.
Seeley EJ, Matthay MA, Wolters PJ (2012) Inflection points in sepsis biology: from local defense to systemic organ injury. Am J Physiol Lung Cell Mol Physiol, 303(5): L355-63.
Singer M, Deutschman CS, Seymour CW et al. (2016) The third international consensus definitions for sepsis and septic shock (Sepsis-3). JAMA, 315(8): 801-10.
Tuinman PR, Dixon B, Levi M et al. (2012) Nebulized anticoagulants for acute lung injury - a systematic review of preclinical and clinical investigations. Crit Care, 16(2): R70.
Vincent JL, Rello J, Marshall J et al. (2009) International study of the prevalence and outcomes of infection in intensive care units. JAMA, 302(21): 2323-9.
Waerhaug K, Kuklin VN, Kirov MY et al. (2008) Recombinant human activated protein C attenuates endotoxin-induced lung injury in awake sheep. Crit Care, 12(4): R104.
Winkler MS, Nierhaus A, Poppe A et al. (2017) Sphingosine-1-phosphate: a potential biomarker and therapeutic target for endothelial dysfunction and sepsis? Shock, 47(6): 666-72.
Yucel G, Zhao Z, El-Battrawy I et al. (2017) Lipopolysaccharides induced inflammatory responses and electrophysiological dysfunctions in human-induced pluripotent stem cell derived cardiomyocytes. Sci Rep, 7(1): 2935.
Ziegler EJ, McCutchan JA, Fierer J et al. (1982) Treatment of gram-negative bacteremia and shock with human antiserum to a mutant Escherichia coli. N Engl J Med, 307(20): 1225-30.
Zurovsky Y, Gispaan I (1995) Antioxidants attenuate endotoxin-induced acute renal failure in rats. Am J Kid Dis, 25(1): 51-7.